The sun's radius (distance from its center to its surface) is about 432,000 miles (695,500 kilometers), approximately 109 times Earth's radius.
The Sun has a temperature of about 5500 degrees C (10,000 degrees F). Temperatures in the sun's core reach over 15 million K.
The energy of the sun comes from nuclear fusion reactions that occur deep inside the sun's core. In a fusion reaction, two atomic nuclei join together, creating a new nucleus. Fusion produces energy by converting nuclear matter into energy.
The sun, like Earth, is magnetic. Scientists describe the magnetism of an object in terms of a magnetic field. This is a region that includes all the space occupied by the object and much of the surrounding space. Physicists define a magnetic field as the region in which a magnetic force could be detected -- as with a compass. Physicists describe how magnetic an object is in terms of field strength. This is a measure of the force that the field would exert on a magnetic object, such as a compass needle. The typical strength of the sun's field is only about twice that of Earth's field.
But the sun's magnetic field becomes highly concentrated in small regions, with strengths up to 3,000 times as great as the typical strength. These regions shape solar matter to create a variety of features on the sun's surface and in its atmosphere, the part that we can see. These features range from relatively cool, dark structures known as sunspots to spectacular eruptions called flares and coronal mass ejections.
Flares are the most violent eruptions in the solar system. Coronal mass ejections, though less violent than flares, involve a tremendous mass (amount of matter). A single ejection can spew approximately 20 billion tons (18 billion metric tons) of matter into space. A cube of lead 3/4 mile (1.2 kilometers) on a side would have about the same mass.
The sun was born about 4.6 billion years ago. It has enough nuclear fuel to remain much as it is for another 5 billion years. Then it will grow to become a type of star called a red giant. Later in the sun's life, it will cast off its outer layers. The remaining core will collapse to become an object called a white dwarf, and will slowly fade. The sun will enter its final phase as a faint, cool object sometimes called a black dwarf.
FATHER SUN
For much of humanity's history on Earth, the sun has been worshipped as a life-giving god which rules the heavens and our solar system. Many a king and queen have claimed to be direct descendants of this all powerful solar deity, the bringer of life.
Ancient peoples and civilizations the world over, from Egypt to the Incas of Peru, erected massive stone temples and monuments which faced the rising sun. They held great religious ceremonies to celebrate the celestial cross: the Winter-Summer Solstice and the Spring-Autumn equinox; which were believed to have cosmic significance.
The equinox means equality, for the day and the nights are of equal length on these ancient Holy days. Rising in a different constellation every 2160 years, it is the sun's changing position on the morning of the equinox, over thousands of years of time, which the ancients believed heralded a new age and the death and birth of the gods. Thus for 2160 years the sun has risen in the houses of Pieces and Virgo during the Spring and Fall Equinox, which is why the Christian god, Jesus, is linked to Virgo, the virgin, and Pieces the two fish. And before Jesus the Pieces there was the god of the Ram, and before the Ram, Taurus the Bull; a new god and a new age every 2160 years, marked by the rising sun in a different constellation on the day of the equinox. And each new god proclaims: thou shalt honor no gods before me.
It is during the equinox that the sun most brilliantly lights up the Earth with dazzling, incredibly bright, multi-colored sheets of light, known as the polar auroras. For millinnea those living in the far northern or southern latitudes have been dazzled by these unearthly solar displays, called the "aurora borealis" in the north, in honor of Aurora, the Roman goddess of the Dawn, and Boreas the Greek god of the wind. In the southern latitudes these ghostly displays of brilliantly colored wavering lights are called the aurora "australis" which is Latin for "south."
The auroras become most beautiful and colorful during the Equinox. Ancient peoples believed these were dancing spirits or the shadows of the gods. In fact, these great shows of light are caused by solar winds as they slam into the Earth's protective magnetic field and the upper atmosphere. The magnetic field is produced by electrical currents generated by the Earth's spinning molten iron core, and extends thousands of miles into space, creating a magnetosphere.
The solar wind, consisting of charged plasma particles emitted by the sun, collides with the charged particles of the Earth's magnetosphere, electrically exciting and interacting with oxygen and nitrogen gasses and ions, creating a rainbow of shimmering greens, reds, purples and blues. For reasons that are not well understood, the solar wind increases in strength and velocity during the equinox, causing massive geomagnetic storms and extraordinarily colorful auroras; though this is not always the case.
From August 28 through September 2, 1859 the Earth was battered by a great magnetic storm triggered by powerful solar winds, solar flares and coronal eruptions from the sun. For five days the planet was enveloped in ghostly shimmering sheets of greens, reds, and blues which were so brilliantly bright that night became day and even the darkest shadows of evening were illuminated with dazzling lights.
THE DEATH OF FATHER SUN
The last breaths of a dying star are exhaled as titanic, planet shaking, solar winds that grow more powerful and destructive with each dying day.
When our own sun begins to die perhaps 6 billion years from now, its solar winds will roll across the Earth like a cosmic tsunami and the night skies will be illuminated for a millennia with fires of light. However, our sun is too small to erupt in a giant supernova, but instead will shrink, then grow, then shrink smaller still until it collapses, implodes and becomes an extremely dense and coldly pale "white dwarf."
However, once our sun begins to die, and well before its final collapse, all intelligent Earthly life will suffocate and drop to the ground gasping for breath. Increasingly powerful solar winds will whip our planet with cosmic hurricane gale force and strip the planet of its atmosphere. The oceans and seas will boil away, and the Earth's atmosphere, heavy with dust and watery mist, will be ripped from its moorings and blown into the black void of deep space. The air literally sucked from their lungs, all animals will collapse, gasping for breath, and die.
But this would not be the end of Earthly life or life on Earth. Trillions of microbes would continue to thrive buried miles beneath the surface of the planet, completely unaffected by this cosmic calamity. Moreover, as the Earth's atmosphere, top soils, and waters are blown into space, trillions of air-born microbes and viral particles would also be jettisoned into the blackness of eternal night. Some of these microbes would form spores and survive in a dormant state, becoming part of the great cosmic sea upon which flow the seeds of life.
A huge nebular cloud would begin to form on the outskirts of the solar system, comprised of dust, debris, frozen water, planetary atmospheres, and the trillions of microbes which had been blown into the far reaches of space. Although the outer layers would be subjected to cosmic radiation and gamma and UV rays, the inner most layers of the cloud would become a protective cocoon.
Much of interstellar space is littered with cosmic dust. These are the remnants of ancient stars and dead planets whose fate, our own planet and sun are destined to replicate. And clinging to these particles of dust and hiding deep within the jagged remnants of innumerable worlds, are countless microbes and viral particle--these are the seeds of life, actual living creatures and their DNA, which flow throughout the cosmos.
SUPERNOVA: THE BEGINNING BEGINS
Life gives birth to life, and stars give birth to stars in an endless cycle of death and rebirth. It's a cosmic dance which may have been ongoing for all eternity.
Birth and death are part of the cycle of life. Some stars give birth as they die; becoming red giants and exploding in a vast supernova, spawning hundreds of infant proto-stars [16,17,18].
These super explosions can light up an entire galaxy. Typically, the explosion creates tremendous shock waves, shattering surrounding planets, and expelling most of the star and remaining planetary debris into the surrounding interstellar medium. This debris eventually becomes part of the surrounding nebular ring created by the solar winds, planetary atmospheres, and expelled mass of the dead star [30,31,32].
Over thousands of years and in response to cosmic shock waves, the debris within these clouds begins to clump together, generating tremendous amounts of energy as they grow larger and denser, until finally they ignite, creating hundreds, even thousands of proto-stars [16-18].
Examples of this cosmic reproductive cycle of death and rebirth are all around us.
An ancient star of Orion grew old, became a red giant, and exploded in a vast supernova millions of years ago, becoming the largest star factory in the heavens [33-35]. Some of these infant stars may be growing planets which may already harbor life. Others are being destroyed, blow torched and burned to death by a blistering flood of ultraviolet radiation from the region's brightest star [36,37].
Thousands of stars were produced by a supernova and nebula in the area of Scorpio which have now spread out over hundreds of light years, migrating across the constellations of Scorpius, Centarus and Crux in the southern skies [38-40]. These are the survivors, formed from the remnants of an ancient parent star whose planets may have also harbored complex life.
Indeed, Dr. Jane Greaves of the University of St. Andrews in Scotland (April , Annual Meeting of the Royal Astronomical Society) has discovered a protoplanet circling a star HL Tau, 520 light-years from Earth. H. L Tau is only 100,000 years old. However, it protoplanet was created just 2,000 years ago and is still growing in size. According to Greaves, in a few hundred thousand years this infant planet could become larger than any in our solar system.
Our sun and the rocky debris which formed the Earth, were produced in an identical fashion [41-44].
NOT ALL RED GIANTS ARE CREATED EQUAL
There are stars twinkling in the darkness of night which are a thousand times larger than our sun. In our own galactic neighborhood these include KW Sagitarii, V354 Cephei, and KY Cygni, each of which is over 1,500 times as large and contain the mass of 150 of our sun. Another heavy weight champion is Eta Carinae which is just 7,500 light years away and weighs over 100 solar masses. It's 4 million times as bright as the Sun. Eta Carinae is the most luminous star we know of and it's extremely hot - 25,000 Kelvin.
VY Canis Majoris, a red hypergiant star in the constellation Canis Major, is more than 2,100 times the size of the Sun. Placed in our Solar System, its surface would extend out past the orbit of Saturn.
The largest stars are the cool supergiants. For example, although over 2000 times as large, VY Canis Majoris is only 3,500 Kelvin, compared with the 5,778 Kelvin of our sun.
Stars lose mass over time, which is blown away by its solar wind. However, as the star begins to die and becomes a red giant, its loses matter at an accelerated rate.
Normally the equilibrium of a star is maintained by the conversion of hydrogen into helium. Within our own sun, 685 million tons of hydrogen is converted to helium each second [23]. The nuclear fusion and conversion of these gasses releases tremendous thermal energy creating an outward pressure which prevents the gravitational collapse and implosion of the star [24,25,26].
As the star grows old the supply of hydrogen fuel begins to dwindle and they cease to generate as much energy. Equilibrium is lost, and the star begins to collapse, making them smaller, but denser, which raises temperatures. In consequence the corona, the crown of the star, begins to expand and the mass of the star is ejected at an accelerated rate in the form of an increasingly dense solar wind.
The super red giant Eta Carinae is so large it casts off 500 times the mass of the Earth every year. Eta Carinae is in its death throes and will soon explode as a supernova. This star and its solar system are already surrounded by a nebular shell comprised of dust, gas, and other material swept into space by its powerful solar wind.
As hydrogen is used up it is converted to helium and eventually a helium core forms in the heart of the dying star. A star become a red giant after it has burned up most of its hydrogen fuel. The loss of expanding thermal energy causes the star to contract and then collapse from its own weight. However, as hydrogen dwindles, the nuclear reactions radiate outward creating an expanding pressure directed toward the upper layers. Simultaneously the core loses support and mass and then collapses even as the surface of the star grows larger in size.
The collapse of the core generates more energy and increases the radiation pressure outward, expanding the star and making it grow increasingly larger in size, becoming a red giant. Thus, the red giant has many times its original size and may have a diameter 10 to 1000 times that of our sun. Luminosities may increase by 1 million times. However, because they are running out of hydrogen fuel, in consequence, they become cooler and may come to have a surface temperature of less than 2000-3000튏, compared with the 5605 Celsius of our sun.
Thus, life on those planets which orbit an expanding star will not necessary be extinguished and burnt completely out of existence. Moreover, when medium mass stars explode, some of their planets may suffer fragmentation but not necessarily obliteration.
Although many times their original size, much of the mass of a red giant is ejected into space long before it explodes. Therefore, although full of sound and fury, when a star explodes, its "bark" may be worse than its bite.
LOW MASS VS HIGH MASS STARS: SUPERNOVA VS WHITE DWARF
Stars the size of our own sun do not explode as supernova or nova unless they have a binary companion. Stars similar to our sun may live 12 or more billion years. When they begin to run out of hydrogen fuel they will quickly lose mass which is ejected into space via the solar wind, forming a nebulae ring. These stars do not explode but instead implode and become a white dwarf surrounded by the ejected gas and dust of the nebula. This will be the fate of our own sun.
The most massive, destructive explosions are produced by stars with initial masses 8 times that of our sun (i.e. 8 or more solar masses). Because these super massive stars consume hydrogen at an accelerated rate, the quickly run out of fuel.
The life times of these super massive stars are relatively brief, around 1 billion years. one billion years is more than enough time for microbes to take root and flourish on any surrounding planets, but not enough time for complex multi-cellular life to develop.
Exploding stars which initially contain 8 or more solar masses may produce a few giant stars or hundreds of small proto stars which may eventually become the size of our own sun.
THE MEDIUM MASS PARENT STAR
Stars which are several times larger than our sun, but less than 8 solar masses may also explode in supernova, though the resulting explosion is much less powerful or dramatic compared to their heavy weight solar cousins. Instead of hundreds of proto stars, they may produce fewer than a dozen.
Some believe that between four to six stars were produced by the supernova which gave rise to our own sun. If true, this indicates that the parent star though certainly many times larger than our sun, was also much less than 8 solar masses.
These less massive stars spend billions of years in a steady state. This is due to the reduced energy requirements as contrasted with stars greater than 8 solar masses.
Given the paucity of evidence for nearby stars the same age as the sun, it could be assumed only a few protostars may have been produced by the supernova and nebular cloud. Thus, the parent star may have been only a few solar masses larger than the sun. This assumption is supported by measurements of the Murchison meteorites which is laden with microfossils and other indices of life.
An analysis of ubiquitous of FE and NI carbides in the rims of magnetite and the carbide grains within the Murchiston meteorite (Brearly 2003) indicates oxidation within the parent body of the meteor, which could have been a planet (Ehrenfreund et al. 2001).
Measuments of silicon carbide (Werner et al. 1994; Nittler & Hoppe, 2005) and presolar SiC grains (Savina et al., 2003) from the Murchison indicates that the grains and silicon are most likely the residue of or were produced secondary to a supernova. An analysis the presolar SiC grains and other isotopes, indicates it was impacted by the supernova of a carbon rich intermediate mass star that was between 1.5 to 3 solar masses (Savina et al., 2003).
Planets have been detected orbiting intermediate mass stars (Lovis and Mayor 2007). Thus, the Murchison may be a remnant of the parent star's solar system, though this can't be determined at this time.
Based on the estimated ages of other intermediate mass stars (Pillitteri and Favata, 2008) it could be assumed the parent star was between 1.5 and 3 solar masses, and at least billion years in age and maybe as many as 6 billion years in age before it entered the red giant phase. Using the Earth as an example, this is also enough time for microbial life to flourish on its planets.
If the parent star was 3 solar masses it may have produced less than 6 stars all similar to our sun. Of course this is only an estimate.
Our Sun is less than 5 billion years old and the Earth is only 4.6 billion years young. Thus, 6 billion years is more than enough time for complex, intelligent life to evolve.
And then after 6 or more billion years and depending on its initial mass, the parent star, like other medium mass stars, began to run out of hydrogen fuel. It then underwent the same dramatic changes as its higher mass cousins.
As it grew in size, losing mass in the process, its stellar wind becames stronger, slamming into surrounding planets, ripping away their atmospheres and enriching the surrounding stellar space with dust and other material which began to congregate at the far edges of the solar system. And not just the atmospheres of its many planets, but trillions of air-borne and surface dwelling microbes were swept away by the solar winds and cast into space.
This would explain why organic material, including Amino acids and over 100 organic molecules have been found in interstellar clouds; organics similar to those produced by life on Earth. These organics include carbon chains and polycyclic aromatic hydrocarbons. on Earth, these same substances were produced biologically for much of the history of this planet.
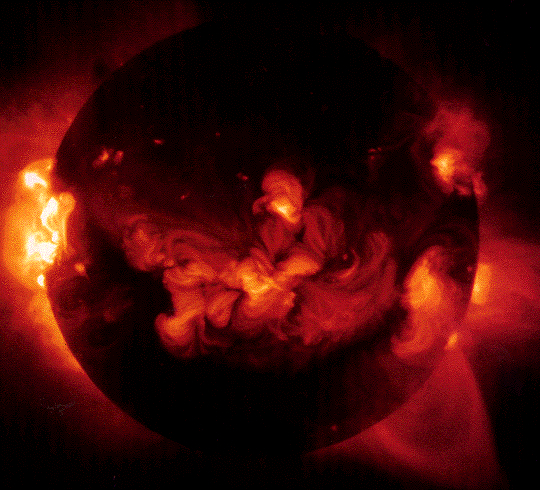
DEATH AND REBIRTH OF THE SUN GODS
Ancient peoples throughout the world worshipped the sun for its life generating powers, believing it a god which governed the cosmic cycle of death and rebirth. In ancient Egypt the morning sun represented rebirth and the god Horus the divine son of Isis and Osiris. The afternoon sun was the God Re, the most powerful of the gods because of his great strength. The redness of the setting sun was the blood of the god as he died. It was the evening sun Atum, the creator god who transported the dead pharoahs to the heavens where they were resurrected and brought back to life to live among the stars which were gods.
The sun also died and was reborn, or so believed the ancients, with death and rebirth taking place during a 3 day period beginning on the Winter Solstice, December 22. "Solstice" means, "Sun standing still" and during the Winter Solstice the sun seems to stop its southerly journey toward the underworld, and then to reverse course, ascending northward into the heavens on December 25--the birthday of innumerable gods and sons of gods. Thus, many ancient cultures celebrated the birth and resurrection of the sun on December 25.
Every son has a father. The ancient Aryans believed the sun was the "son" of the Sky God, and was thus the "Son of the sky" and had been created and fathered by the Heavenly Father who in turn had been sired by Father Sun. The son of the Sun-God becomes God the Sun. Christians borrowing from the ancient pagans, believed Jesus to be the "son of god" and "god the son" the incarnation of the Heavenly father, and who dies and is resurrected, ascending to heaven, becoming one with god.
Thus ancient peoples throughout the world believed the "Son of the Sun-God" was sired by God the Sun, becoming the Sun God who sets and dies and ascends to heaven, is resurrected and reborn to become the Heavenly Father, the Sun, in an endless cycle of death and rebirth, thus guaranteeing eternal life to all god's children--so claim the ancients.
THE BIRTH OF THE SUN & SOLAR SYSTEM
Five billion years ago, a giant star, likely ringed with planets some just like our own, exploded in a vast supernova, giving birth to less than a dozen new proto stars, including our own Sun. These solar siblings may have included up to 6 sister stars, such as "18 Scorpii" which is nearly identical, and close to the same age as our Sun. "18 Scorpii" dwells in the constellation of Scorpius, just 46 light years away. The Sun and "18 Scorpii" could be twins.
Consider, the Sun weighs nearly 2.2 billion billion billion tons and is 865,000 miles in diameter. Over 1 million Earths could easily fit inside the Sun.
18 Scorpii is perhaps a billion tons more massive, and like our Sun, about 880,000 miles in diameter.
Like the Earth and the other planets, the Sun rotates on its axis. It completes a 360 degree spin once every 26 days whereas 18 Scorpii takes 23.
The temperature of our bright yellow sun is 9,940 scorching degrees Fahrenheit compared to 9,960 degrees on 18 Scorpii. The temperatures of the sun are effected by intense magnetic activity which causes blemishes to break out on the surface. These are known as sunspots, dark splotches on the face of the sun which are much cooler than the surrounding surface. When these black splotches group together, solar flares erupt and coronal masses are ejected from the neighboring areas. Sunspots occur in cycles, waxing and waning every 11 years on our sun, and around 11 years for 18 Scorpii.
Other possible solar sisters include HIP 56948. In fact, the Catalog of Nearby Habitable Systems developed by scientists Jill Tarter and Margaret Turnbull, includes 18 Scorpii and HIP 56948, as well as over 118,216 additional stars, that may be orbited by living planets. And like our sun, these stars were created from the remnants of an ancient supernova.
When our ancient, parent star exploded, its planets were baked, fried, burned, broken, and blasted apart into gas, dust, and moon-sized islands of debris. Shock waves and the force of the explosion cast the remnants of this ancient star and its planetary system into the chasms of space. Much of this debris became part of the giant swirling, spinning, expanding nebular cloud on the outskirts of the solar system, already created by the solar wind. This expanding shell consisted of dust, microbes, viral particles, spores, oceans of ice, shattered planets, and comets, asteroids and meteors hundreds, even thousands of miles in size [45,46].
Scientific studies have determined that the outer regions of these nebular clouds is very cold and low in pressure and subject to bombardment by UV and gamma rays (B. Fegley, Space Science Review, 1999, 90, 239). However, the inner central most layers cannot be penetrated by gamma and cosmic rays, and are much warmer and thus provides a protective cocoon for those creatures dwelling within.
Within a few million years these titanic molecular clouds begin to condense, collide, and clump together, forming planetary nebula, also known as "cometary knots" due to their resemblance to planet-size comets [47,48; (P. Ehrenfreund & K. M. Menten, 2002. From Molecular Cluds to the Origin of Life. In G. Horneck & C. Baumstark-Khan. Astrobiology, Springer, 2002).]. These planet-sized knots of clumpy matter are in motion and begin to rotate faster and faster, becoming hotter and growing denser and larger in size as debris slamms into it.
Because of gravitational pressures and cosmic shock waves, eventually these planetary nebula completely collapse and then ignite, creating proto-stars. This is how our own sun was born. The nebular remnants of the parent star formed perhaps a dozen bright burning proto-stars surrounded by clouds of gas, dust, oceans of frozen water, and mountains of stellar debris thousands of miles in size. Not all survived.
Within a brief moment of time, ranging from 100,000 years to 50 million years [50-54, Greaves et al], the pressure and density of hydrogen in the centre of each proto star became great enough and temperatures hot enough that it triggered a thermonuclear reaction, with the exploding, expanding thermal energy countering the gravitational forces of contraction thereby creating equilibrium and a full blown star, our sun [55,56].
Thus, our sun was born among a cluster of sister stars some of which survived and are now likely ringed with planets just like our own.
BIRTH OF THE PLANETS
As thermonuclear equilibrium was established giving stability to our sun, the remaining nebular clouds of gas, hydrogen, dust, heavy metals, oceans of frozen water, and giant masses of rocky planetary debris, continued to spin and began clumping and collapsing together. Thus a proto-planetary disk formed from the remnants of the dead parent star and its broken, shattered planets, and began to flatten out and orbit in the same direction around the sun [57,58,59].
A solar wind began to blow from the new born sun, dispersing the remaining, lighter gaseous elements toward the outer edges of the solar-proto-planetary disk [60,61]. Debris with the highest melting points, such as silicates and metals, formed the inner rims of the planetary disk, congregating together to create the rocky, heavy metal inner terrestrial planets including the Earth [62,63].
Thus, the Earth is comprised of the shattered remnants of the parent star and its rocky inner planets and was born amongst microbe-hugging debris which formed the initial nebular ring and then gave rise to the solar disc.
By contrast, water, gasses and lighter elements and compounds were more resistant to the gravitational effects of the still growing sun, but more susceptible to its heat and the solar wind which swept through the infant solar system. Thus these volatile icy compounds and incredibly toxic, poisonous gasses were blown far away and remained at a greater distance from the sun, eventually freezing and becoming part of the big gaseous planets Jupiter and Saturn, and the ice-giants Neptune and Uranus [64-68].
And yet, despite this violent activity, innumerable microbes would have easily survived. A protoplanetary disc consists of layers. Like the nebular cloud, the outermost layer is subjected to gamma and cosmic rays and radiation from the sun. However, the central most layer cannot be penetrated by these deadly rays or UV photons (P. Ehrenfreund & K. M. Menten, 2002. From Molecular Cluds to the Origin of Life. In G. Horneck & C. Baumstark-Khan. Astrobiology, Springer, 2002), and thus provided a protective environment for microbial survivors.
Planet formation proceeds at a relatively rapid pace, from a few hundred years for a small rocky planet the size of the Earth to one million years for Jupiter and Saturn-sized gas giants [69-72].
Thus, around 4.6 billion years ago, the Earth and the other planets were formed from the remnants of the dead parent star, and began to orbit in the same direction around the sun.
Computer models and observations of planet-formation have given us a clear understanding of the mechanisms involved [64-75]. Within just a few thousand years the remnants of the supernova and the nebular cloud which surrounded our new sun, began to flatten out into a swirling circular proto planetary disk. After just a few spins around the new proto-star, islands of debris began to collide and clump together forming jagged moon-like planets increasing in size by accretion as they orbited our sun.
According to the most conservative estimates, if mechanisms of accretion are very slow, it could take up to a million years for a massive solid planetary core to form. Then it would quickly snow ball in size through clumping and as debris continued to crash into it [72-75].
Based on more liberal interpretations, these planetary cores may form in a few thousand years (Greaves, et al.) Greaves has discovered a protoplanet that was created within 2,000 years ago, circling a infant star HL Tau, which is just 100,000 years old.
However, these cores may have been present even before our sun was born. Rather than initiated via accretion, they may have been comprised of the broken remnants of those worlds which originally orbited the parent star.
Because most of the parent star's mass had been lost before the explosion, the force and destructive power had been greatly reduced and many of its planets although broken or shattered, may have been left largely intact. When the parent star exploded and it's gravitational influences were eliminated, these broken worlds would have been sent tumbling into the the wilds of space, joining the growing nebular cloud, or they may have begun drifting until captured by the gravitational forces of a distant solar system or a new and growing star.
Indeed, studies have shown that giant planets orbiting distant stars slowly migrate toward the inner solar system (S. Udry & M. Mayor, The Diversity of Extrasolar planets around solar type stars, In G. Horneck & C. Baumstark-Khan. Astrobiology, Springer, 2002). Thus if the mass of the star is suddenly lost, they will migrate outward.
Planets can perturb one another and can eject them from the solar system (S. Udry & M. Mayor, The Diversity of Extrasolar planets around solar type stars, In G. Horneck & C. Baumstark-Khan. Astrobiology, Springer, 2002). Without a giant sun at the center, smaller planets may be ejected by larger planets, only to join a newly forming solar system millions of years later.
This may explain why many of the planets of this solar system were struck by wayward worlds which crashed into them during the early years of solar system formation. For example, around 4 billion years ago, a Mars-sized planet hit the Earth with so much force that the ejected mass came to form the moon.
Likewise, around 4 billion years ago, the northern plains of Mars was gutted by a planet-sized body which left an elliptical depression 6,600 miles long and 4,000 miles wide. The planet Mercury also suffered a collision which left a titanic impact basis. And the same is true of Uranus which was struck so hard it was knocked on its side leaving its rotation axis tilted at an extreme angle. Thus, there is considerable evidence that planet-sized objects were careening through the solar system during its early stages of formation.
Nevertheless, even based on conservative estimates which do not take into account the presence of largely intact planetary bodies, it is clear that multiple planets can form in a relatively short space of time, within the first million years after the birth of the sun.
Hundreds of planets have now been detected orbiting distant stars [76-77] which suggests similar mechanisms of planet and star formation take place throughout the cosmos in less than a million years and even within a few hundred thousand years.
A million years is more than enough time for any surviving life forms contained in the remnants of the parent star's shattered planets to find safe harbor within a new world made up of this debris. Some microbes become dormant and can rewaken even after 250 million years [78-80]. In fact, only one microbe had to survive, and once on Earth, or another suitable world, could cover the planet in bacterial offspring within a few months.
Mass and density
The sun has 99.8 percent of the mass in the solar system. The sun's mass is roughly 2 X 1027 tons. This number would be written out as a 2 followed by 27 zeros. The sun is 333,000 times as massive as Earth. The sun's average density is about 90 pounds per cubic foot (1.4 grams per cubic centimeter). This is about 1.4 times the density of water and less than one-third of Earth's average density.
Composition
The sun, like most other stars, is made up mostly of atoms of the chemical element hydrogen. The second most plentiful element in the sun is helium, and almost all the remaining matter consists of atoms of seven other elements. For every 1 million atoms of hydrogen in the entire sun, there are 98,000 atoms of helium, 850 of oxygen, 360 of carbon, 120 of neon, 110 of nitrogen, 40 of magnesium, 35 of iron, and 35 of silicon. So about 94 percent of the atoms are hydrogen, and 0.1 percent are elements other than hydrogen and helium.
But hydrogen is the lightest of all elements, and so it accounts for only about 72 percent of the mass. Helium makes up around 26 percent.
The inside of the sun and most of its atmosphere consist of plasma. Plasma is basically a gas whose temperature has been raised to such a high level that it becomes sensitive to magnetism. Scientists sometimes emphasize the difference in behavior between plasma and other gas. They say that plasma is a fourth state of matter, alongside solid, liquid, and gas. But in general, scientists make the distinction between plasma and gas only when technically necessary.
The relative amounts of plasma and other gas in a given part of the solar atmosphere depends on the temperature. As the temperature increases, more and more atoms become ionized, and the atoms that are ionized lose more and more electrons. The highest part of the solar atmosphere, called the corona, is strongly ionized. The corona's temperature is usually about 3 million to 5 million K, more than enough to strip away over half the 26 electrons in its iron atoms.
How much of a gas is made up of single atoms and how much of molecules also depends upon its temperature. If the gas is relatively hot, the atoms will move about independently. But if the gas is relatively cool, its atoms may bond (combine chemically), creating molecules. Much of the sun's surface consists of a gas of single atoms. But sunspots are so cool that some of their atoms can bond to form molecules.
The remainder of this article follows the general practice of scientists by referring to both plasma and other gas simply as gas.
Energy output
Most of the energy emitted (sent out) by the sun is visible light and a related form of radiation known as infrared rays, which we feel as heat. Visible light and infrared rays are two forms of electromagnetic radiation. The sun also emits particle radiation, made up mostly of protons and electrons.
Electromagnetic radiation
Electromagnetic radiation consists of electrical and magnetic energy. The radiation can be thought of as waves of energy or as particle-like "packets" of energy called photons.
Visible light, infrared rays, and other forms of electromagnetic radiation differ in their energy. Six bands of energy span the entire spectrum (range) of electromagnetic energy. From the least energetic to the most energetic, they are: radio waves, infrared rays, visible light, ultraviolet rays, X rays, and gamma rays. Microwaves, which are high-energy radio waves, are sometimes considered to be a separate band. The sun emits radiation of each type in the spectrum.
The amount of energy in electromagnetic waves is directly related to their wavelength, the distance between successive wave crests. The more energetic the radiation, the shorter the wavelength. For example, gamma rays have shorter wavelengths than radio waves. The energy in an individual photon is related to the position of the photon in the spectrum. For instance, a gamma ray photon has more energy than a photon of radio energy.
All forms of electromagnetic radiation travel through space at the same speed, commonly known as the speed of light: 186,282 miles (299,792 kilometers) per second. At this rate, a photon emitted by the sun takes only about 8 minutes to reach Earth.
The essential difference between plasma and other gas is an effect of the temperature increase: This increase has made the gas atoms come apart. What is left -- the plasma -- consists of electrically charged atoms called ions and electrically charged particles called electrons that move about independently.
An electrically neutral atom contains one or more electrons that act as though they form a shell or shells around its central region, its nucleus. Each electron carries a single unit of negative electric charge. Deep inside the atom is the nucleus, which has almost all the atom's mass. The simplest nucleus, that of the most common form of hydrogen, consists of a single particle known as a proton. A proton carries a single unit of positive electric charge. All other nuclei have one or more protons and one or more neutrons. A neutron carries no net charge, and so every nucleus is electrically positive. But a neutral atom has as many electrons as protons. The net electric charge of a neutral atom is therefore zero.
An atom or molecule that comes apart by losing one or more electrons has a positive charge and is called an ion or, sometimes, a positive ion. Most of the atoms inside the sun are positive ions of the most common form of hydrogen. Thus, most of the sun consists of single protons and independent electrons.
The amount of electromagnetic radiation from the sun that reaches the top of Earth's atmosphere is known as the solar constant. This amount is about 1,370 watts per square meter. But only about 40 percent of the energy in this radiation reaches Earth's surface. The atmosphere blocks some of the visible and infrared radiation, almost all the ultraviolet rays, and all the X rays and gamma rays. But nearly all the radio energy reaches Earth's surface.
Particle radiation
Protons and electrons flow continually outward from the sun in all directions as the solar wind. These particles come close to Earth, but Earth's magnetic field prevents them from reaching the surface.
However, more intense concentrations of particles from flares and coronal mass ejections on the sun reach Earth's atmosphere. These particles are known as solar cosmic rays. Most of them are protons, but they also include heavier nuclei as well as electrons. They are extremely energetic. As a result, they can be hazardous to astronauts in orbit or to orbiting satellites.
The cosmic rays cannot reach Earth's surface. When they collide with atoms at the top of the atmosphere, they change into a shower of less energetic particles. But, because the solar events are so energetic, they can create geomagnetic storms, major disturbances in Earth's magnetic field. The storms, in turn, can disrupt electrical equipment on Earth's surface. For example, they can overload power lines, leading to blackouts.
Color
In the visible-light band of the electromagnetic spectrum are all the colors of the rainbow. Sunlight consists of all these colors. Most of the sun's radiation comes to us in the yellow-green part of the visible spectrum. However, sunlight is white. When the atmosphere acts as a filter for the setting sun, the sun may look yellow or orange.
You can view the colors in sunlight by using a prism to separate and spread them out. Red light, which is produced by the radiation with the least energy per photon -- and the longest waves -- will be at one end of the spectrum. The red light will gradually shade into orange light, which, in turn, will shade into yellow light. Next to yellow will be green, and then will come blue. In some lists of the colors of the rainbow, indigo comes after blue. The last color will be violet, produced by the radiation with the most energy per photon -- and the shortest waves. Such color listings are not meant to indicate that sunlight has only six or seven colors. Each shading is itself a color. Nature produces many more colors than people have ever named.
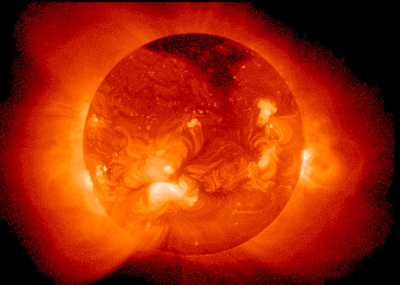
Rotation
The sun makes a complete rotation in about a month. But because the sun is a gaseous body rather than a solid one, different parts of the sun rotate at different rates. Gas near the sun's equator takes about 25 days to rotate once, while gas at higher latitudes may take slightly more than 28 days. The sun's axis of rotation is tilted by a few degrees from the axis of Earth's orbit. Thus, either the sun's north geographic pole or its south geographic pole is usually visible from Earth.
Vibration
The sun vibrates like a bell that is continually struck. But the sun produces more than 10 million individual "tones" at the same time. The vibrations of the solar gas are mechanically similar to the vibrations of air -- also a gas -- that we know as sound waves. Astronomers therefore refer to the solar waves as sound waves, though the vibrations are much too slow for us to hear. The fastest solar vibrations have a period of about 2 minutes. A vibration's period is the amount of time taken for a complete cycle of vibration -- one back-and-forth movement of the vibrating object. The slowest vibration that a human being can hear has a period of about 1/20 of a second.
Most of the sun's sound waves originate in convection cells -- large concentrations, or clumps, of gas beneath the surface. These cells carry energy to the surface by rising, just as water boiling in a pan rises to the surface. The word convection refers to the boiling motions of the cells. As the cells rise, they cool. They then fall back down to the level at which the upward motion started. As the cells fall, they vibrate violently. The vibrations cause sound waves to move out from the cells.
Because the sun's atmosphere has so little mass, sound waves cannot travel through it. Therefore, when a wave reaches the surface, it turns back inward. As a result, a bit of the surface bobs up and down. As the wave travels inward, it begins to curve back toward the surface. The amount by which it curves depends on the density of the gas through which it travels and other factors. Eventually, the wave reaches the surface and turns inward again. It continues to travel until it loses all its energy to the surrounding gas.
The waves that travel downward the greatest distance have the longest periods. Some of these waves approach the sun's core and have periods of several hours.
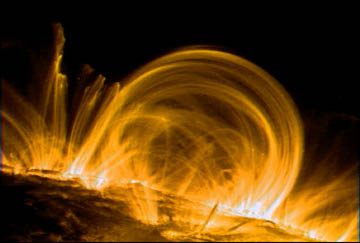
Magnetic field
Some of the time, the sun's magnetic field has a simple overall shape. At other times, the field is extremely complex. The simple field resembles the field that would be present if the sun's axis of rotation were a huge bar magnet. You can see the shape of a bar magnet's field by conducting an experiment with iron filings. Place a sheet of paper on a bar magnet and then sprinkle iron filings on the paper. The filings will form a pattern that reveals the shape of the magnetic field. Many of the filings will gather in D-shaped loops that connect the ends of the magnet.
Physicists define the field in terms of imaginary lines that give rise to the loops of filings. These lines are called field lines, flux lines, or lines of force. Scientists assign these lines a direction, and the bar magnet is said to have a magnetic north pole at one end and a magnetic south pole at the other end. The field lines go out of the magnet from the north pole, loop around, and return to the magnet at the south pole.
The cause of the sun's magnetic field is, in part, the movement of the convection cells. Any electrically charged object can create a magnetic field simply by moving. The convection cells, which are composed of positive ions and electrons, circulate in a way that helps create the solar field.
When the sun's magnetic field becomes complex, field lines resemble a kinked, twisted garden hose. The field develops kinks and twists for two reasons: (1) The sun rotates more rapidly at the equator than at higher latitudes, and (2) the inner parts of the sun rotate more rapidly than the surface. The differences in rotational speed stretch field lines in an easterly direction. Eventually, the lines become so distorted that the kinks and twists develop.
In some areas, the field is thousands of times stronger than the overall magnetic field. In these places, clusters of field lines break through the surface, creating loops in the solar atmosphere. At one end of the loop, the breakthrough point is a magnetic north pole. At this point, the direction of the field lines is upward -- that is, away from the interior. At the other end of the loop, the breakthrough point is a magnetic south pole, and the lines point downward. A sunspot forms at each point. The field lines guide ions and electrons into the space above the sunspots, producing gigantic loops of gas.
The number of sunspots on the sun depends on the amount of distortion in the field. The change in this number, from a minimum to a maximum and back to a minimum, is known as the sunspot cycle. The average period of the sunspot cycle is about 11 years.
At the end of a sunspot cycle, the magnetic field quickly reverses its polarity and loses most of its distortion. Suppose the sun's magnetic north pole and its geographic north pole were at the same place at the start of a given cycle. At the beginning of the next cycle, the magnetic north pole would be at the same place as the geographic south pole. A change of polarity from one orientation to the other and back again equals the periods of two successive sunspot cycles and is therefore about 22 years.
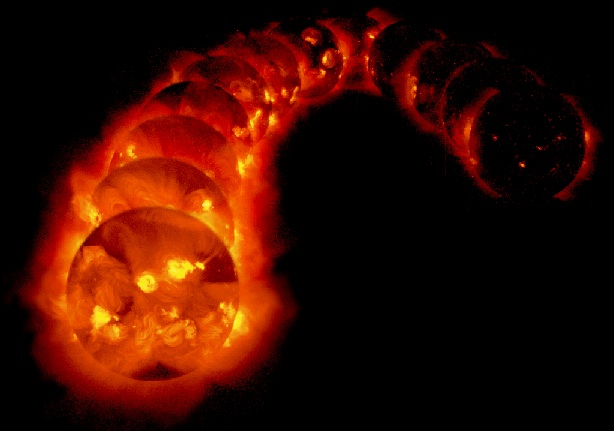
Nuclear fusion
Nuclear fusion can occur in the core of the sun because the core is tremendously hot and dense. Because nuclei have a positive charge, they tend to repel one another. But the core's temperature and density are high enough to force nuclei together.
The most common fusion process in the sun is called the proton-proton chain. This process begins when nuclei of the simplest form of hydrogen -- single protons -- are forced together one at a time. First, a nucleus with two particles forms, then a nucleus with three particles, and finally a nucleus with four particles. The process also produces an electrically neutral particle called a neutrino.
The final nucleus consists of two protons and two neutrons, a nucleus of the most common form of helium. The mass of this nucleus is slightly less than the mass of the four protons from which it forms. The lost mass is converted into energy. The amount of energy can be calculated from the German-born physicist Albert Einstein's famous equation E = mc-squared (E=mc2). In this equation, the symbol E represents the energy, m the mass that is covered, and c-squared (c2) the speed of light multiplied by itself.
Comparison With Other Stars
Fewer than 5 percent of the stars in the Milky Way are brighter or more massive than the sun. But some stars are more than 100,000 times as bright as the sun, and some have as much as 100 times the sun's mass. At the other extreme, some stars are less than 1/10,000 as bright as the sun, and a star can have as little as 7/100 of the sun's mass. There are hotter stars, which are much bluer than the sun; and cooler stars, which are much redder.
The sun is a relatively young star, a member of a generation of stars known as Population I stars. An older generation of stars is called Population II. There may have existed an earlier generation, called Population III. However, no members of this generation are known. The remainder of this section refers to three generations of stars.
The three generations differ in their content of chemical elements heavier than helium. First-generation stars have the lowest percentage of these elements, and second-generation stars have a higher percentage. The sun and other third-generation stars have the highest percentage of elements heavier than helium.
The percentages differ in this way because first- and second-generation stars that "died" passed along their heavier elements. Many of these stars produced successively heavier elements by means of fusion in and near their cores. The heaviest elements were created when the most massive stars exploded as supernovae. Supernovae enrich the clouds of gas and dust from which other stars form. Other sources of enrichment are planetary nebulae, the cast-off outer layers of less massive stars.
Zones of the Sun
The sun and its atmosphere consist of several zones or layers. From the inside out, the solar interior consists of the core, the radiative zone, and the convection zone. The solar atmosphere is made up of the photosphere, the chromosphere, a transition region, and the corona. Beyond the corona is the solar wind, which is actually an outward flow of coronal gas.
Because astronomers cannot see inside the sun, they have learned about the solar interior indirectly. Part of their knowledge is based on the observed properties of the sun as a whole. Some of it is based on calculations that produce phenomena in the observable zones.
Core
The core extends from the center of the sun about one-fourth of the way to the surface. The core has about 2 percent of the sun's volume, but it contains almost half the sun's mass. Its maximum temperature is over 15 million Kelvins. Its density reaches 150 grams per cubic centimeter, nearly 15 times the density of lead.
The high temperature and density of the core result in immense pressure, about 200 billion times Earth's atmospheric pressure at sea level. The core's pressure supports all the overlying gas, preventing the sun from collapsing.
Almost all the fusion in the sun takes place in the core. Like the rest of the sun, the core's initial composition, by mass, was 72 percent hydrogen, 26 percent helium, and 2 percent heavier elements. Nuclear fusion has gradually changed the core's contents. Hydrogen now makes up about 35 percent of the mass in the center of the core and 65 percent at its outer boundary.
Radiative Zone
Surrounding the core is a huge spherical shell known as the radiative zone. The outer boundary of this zone is 70 percent of the way to the solar surface. The radiative zone makes up 32 percent of the sun's volume and 48 percent of its mass.
The radiative zone gets its name from the fact that energy travels through it mainly by radiation. Photons emerging from the core pass through stable layers of gas. But they scatter from the dense particles of gas so often that an individual photon may take 1,000,000 years to pass through the zone.
At the bottom of the radiative zone, the density is 22 grams per cubic centimeter -- about twice that of lead -- and the temperature is 8 million K. At the top of the zone, the density is 0.2 gram per cubic centimeter, and the temperature is 2 million K.
The composition of the radiative zone has remained much the same since the sun's birth. The percentages of the elements are nearly the same from the top of the radiative zone to the solar surface.
Convection Zone
The highest level of the solar interior, the convection zone, extends from the radiative zone to the sun's surface. This zone consists of the "boiling" convection cells. It makes up about 66 percent of the sun's volume but only slightly more than 2 percent of its mass. At the top of the zone, the density is near zero, and the temperature is about 5800 K. The convection cells "boil" to the surface because photons that spread outward from the radiative zone heat them.
Astronomers have observed two main kinds of convection cells -- (1) granulation and (2) supergranulation. Granulation cells are about 600 miles (1,000 kilometers) across. Supergranulation cells reach a diameter of about 20,000 miles (30,000 kilometers).
Photosphere
The lowest layer of the atmosphere is called the photosphere. This zone emits the light that we see. The photosphere is about 300 miles (500 kilometers) thick. But most of the light that we see comes from its lowest part, which is only about 100 miles (150 kilometers) thick. Astronomers often refer to this part as the sun's surface. At the bottom of the photosphere, the temperature is 6400 K, while it is 4400 K at the top.
The photosphere consists of numerous granules, which are the tops of granulation cells. A typical granule exists for 15 to 20 minutes. The average density of the photosphere is less than one-millionth of a gram per cubic centimeter. This may seem to be an extremely low density, but there are tens of trillions to hundreds of trillions of individual particles in each cubic centimeter.
Chromosphere
The next zone up is the chromosphere. The main characteristic of this zone is a rise in temperature, which reaches about 10,000 K in some places and 20,000 K in others.
Astronomers first detected the chromosphere's spectrum during total eclipses of the sun. The spectrum is visible after the moon covers the photosphere, but before it covers the chromosphere. This period lasts only a few seconds. The emission lines in the spectrum seem to flash suddenly into visibility, so the spectrum is known as the flash spectrum.
The chromosphere is apparently made up entirely of spike-shaped structures called spicules (SPIHK yoolz). A typical spicule is about 600 miles (1,000 kilometers) across and up to 6,000 miles (10,000 kilometers) high. The density of the chromosphere is about 10 billion to 100 billion particles per cubic centimeter.
Transition Region
The temperature of the chromosphere ranges to about 20,000 K, and the corona is hotter than 500,000 K. Between the two zones is a region of intermediate temperatures known as the chromosphere-corona transition region, or simply the transition region. The transition region receives much of its energy from the overlying corona. The region emits most of its light in the ultraviolet spectrum.
The thickness of the transition region is a few hundred to a few thousand miles or kilometers. In some places, relatively cool spicules extend from the chromosphere high into the solar atmosphere. Nearby may be areas where thin, hot coronal structures reach down close to the photosphere.
Corona
Corona is the part of the sun's atmosphere whose temperature is greater than 500,000 K. The corona consists of such structures as loops and streams of ionized gas. The structures connect vertically to the solar surface, and magnetic fields that emerge from inside the sun shape them. The temperature of a given structure varies along each field line. Near the surface, the temperature is typical of the photosphere. At higher levels, the temperature has chromospheric values, then values of the transition region, then coronal values.
In the part of the corona nearest the solar surface, the temperature is about 1 million to 6 million K, and the density is about 100 million to 1 billion particles per cubic centimeter. The temperature reaches tens of millions of Kelvins when a flare occurs.
Solar Wind
The corona is so hot that it extends far into space and continually expands. The flow of coronal gas into space is known as the solar wind. At the distance of Earth from the sun, the density of the solar wind is about 10 to 100 particles per cubic centimeter.
The solar wind extends far into interplanetary space as a large, teardrop-shaped cavity called the heliosphere. The sun and all the planets are inside the heliosphere. Far beyond the orbit of Pluto, the farthest planet, the heliosphere joins the interstellar medium, the dust and gas that occupy the space between the stars.
Solar Activity
The sun's magnetic fields rise through the convection zone and erupt through the photosphere into the chromosphere and corona. The eruptions lead to solar activity, which includes such phenomena as sunspots, flares, and coronal mass ejections. Areas where sunspots or eruptions occur are known as active regions. The amount of activity varies from a solar minimum at the beginning of a sunspot cycle to a solar maximum about 5 years later. The number of sunspots that exist at a given time varies. on the side of the solar disk that we see, this number ranges from none to approximately 250 individual sunspots and clusters of sunspots.
Sunspots
Sunspots are dark, often roughly circular features on the solar surface. They form where denser bundles of magnetic field lines from the solar interior break through the surface.
'연구하는 인생 > Nature·Universe' 카테고리의 다른 글
MOON (0) | 2009.12.05 |
---|---|
DARK ENERGY & THE EXPANDING, ACCELERATING UNIVERSE? (0) | 2009.12.05 |
Life has existed on the MOON & MARS (0) | 2009.12.04 |
MOON - photo (0) | 2009.11.30 |
MOON (0) | 2009.11.29 |